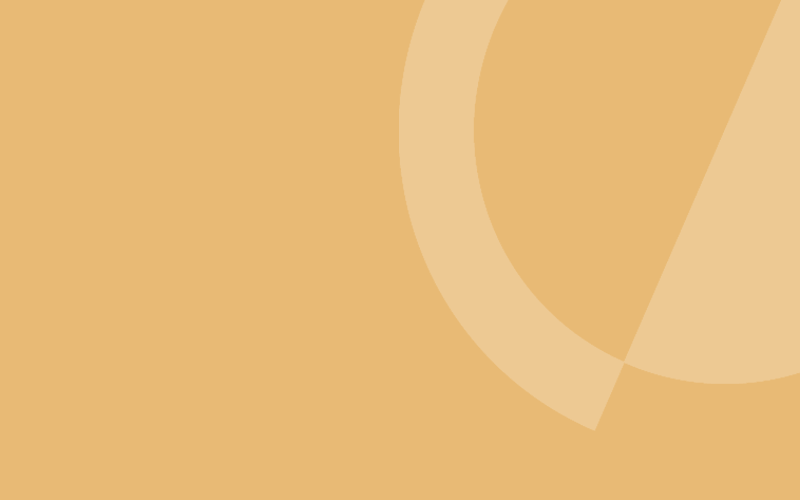
5 August 2021
Life cycle Analysis

Making changes to our road transport sector, including its decarbonisation, is incredibly urgent and important in the race to tackle climate change, with about 27% of UK greenhouse gas (GHG) emissions currently coming from this sector. Not only that, but for the benefit of the population’s health, there is a need to improve air quality in our towns and cities, where road transport emissions are one of the biggest contributors to localised pollution.
Various solutions are being proposed, and these vary by sector, but are often only focused on taking a very restricted view of the issues.
Life-cycle analysis
At Coryton, our approach is much more holistic. Initially we focused on climate change and consequently, have analysed each sector and looked at the probable impact on life-cycle greenhouse gas (GHG) emissions of the relevant propulsion systems and associated energy vectors. Life cycle analysis is a technique for quantifying the environmental and human health impacts of a product over its lifespan, and is sometimes referred to as ‘cradle-to-grave analysis’.
In figure 1, you’ll see how the various parts of a product’s lifecycle fit together. It’s important we consider all these steps to avoid unforeseen consequences that could be caused by focusing on only one part. While this is shown for a passenger car, the principle applies to all products.
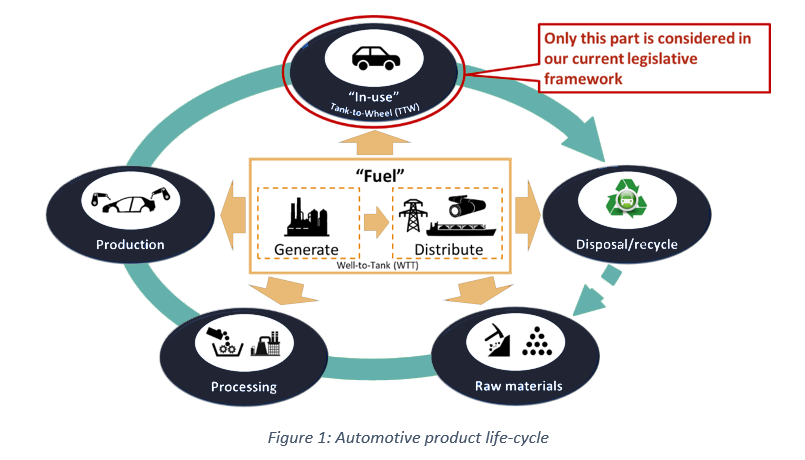
Life cycle analysis (LCA) considers the impact of each step on the particular variable under consideration, in our case GHG emissions. GHG emissions are usually quantified in terms of equivalent CO2. While there are many emissions that impact climate change (e.g. methane), in LCA they are each converted to an equivalent amount of CO2 and then summed to calculate the total in terms of CO2 equivalent or CO2e.
LCA considers the CO2 emissions associated with, for example, mining the raw materials (e.g. iron ore), processing those into usable material (e.g. rolls of sheet steel), manufacturing the product (e.g. a car), the use of the product during its lifetime which needs to include any “fuel” that is used, and then the disposal/recycling of that product at the end of its life.
Currently we only consider tailpipe emissions in our legislative framework i.e. those that are emitted from the exhaust pipe of each individual vehicle, often referred to as “tank-to-wheels” (TTW). From this perspective, it’s clear to see why the focus is on electric and fuel cell vehicles – they emit no CO2 from the vehicle at the point of use, so-called “zero emission vehicles”. Although they are technically zero tailpipe emission vehicles, this neglects a significant proportion of the full life cycle emissions of these vehicles and the “zero emission” moniker is very misleading.
Consequently, the move to electric propulsion for everything is not the panacea it might seem and, sadly, there is no “one-size-fits-all” solution.
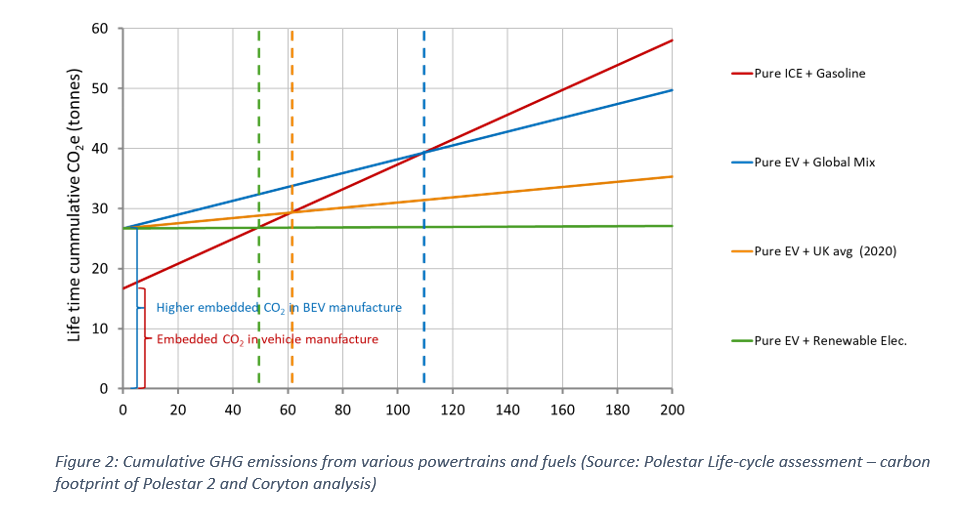
For example, Polestar (a manufacturer of pure electric vehicles) recently published an LCA analysis for the Polestar 2 vehicle compared to a Volvo XC40 (Figure 2). They estimated the crossover point to be anywhere between 50,000 and 112,000km, meaning you need to drive the electric vehicle this distance before you have “paid back” the front-loaded CO2 emissions in the manufacturing process, with the CO2 reduction from using electricity rather than a fossil fuel. The range of payback distances depends on the carbon intensity (gCO2e/kWh) of electricity grid used for charging, in this case the lower end for wind (i.e. 100% renewable electricity) and the higher end for a global average.
However, as is common in these types of analysis, the effect of hybridisation and sustainable fuels are conspicuously omitted. Sustainable fuels are a carbon neutral source of energy (utilising carbon captured from the atmosphere or recovered from waste feedstocks), that are economically viable and socially acceptable to end users. They can come from a variety of sources/processes including biofuels, synthetic fuels and e-fuels. If we perform further analysis and include these effects we get the results shown in Figure 3.
Simply by running the conventional ICE vehicle on a 100% sustainable fuel (in this case our 95RON drop-in biogasoline which offers >80% (RED II) GHG savings over a conventional fossil fuel), the CO2 emissions after 200,000km are within <20% of those of a BEV running on 100% renewable electricity.
If the powertrain is assumed to be a plug-in hybrid, firstly the embedded emissions are reduced due to the smaller battery pack (100km range). If this vehicle is then charged with 100% renewable electricity when plugged in, the CO2 emissions after 200,000km are a little more than the BEV and the conventional ICE on sustainable fuels. If we now allow the PHEV to run on sustainable fuel as well, the CO2 emissions after 200,000km are less than 7.5% more than the pure BEV running on 100% renewable electricity and substantially less than a BEV recharging on the UK grid.
Many researchers dismiss this approach, claiming that there is not enough biomass available in order to replace all the fossil fuel available on our forecourts but this misses the fact that we do not need to replace it all in one go. The petrol available at the pump is already a blend of many components and it’s perfectly feasible to start replacing more of the fossil-components with sustainable components.
To some extent this has already started. The petrol at the pump can currently include up to 5% bioethanol (E5) and this is set to increase to 10% (E10) for the standard grade, 95RON fuel. But we can go further than that by replacing more of the remaining 90% fossil gasoline with bio-gasoline. Even at a level of 10% bio-gasoline, this would result in 20% of our fuel coming from renewable sources, and we can ramp that up over time as incentives and investments scale up the industry.
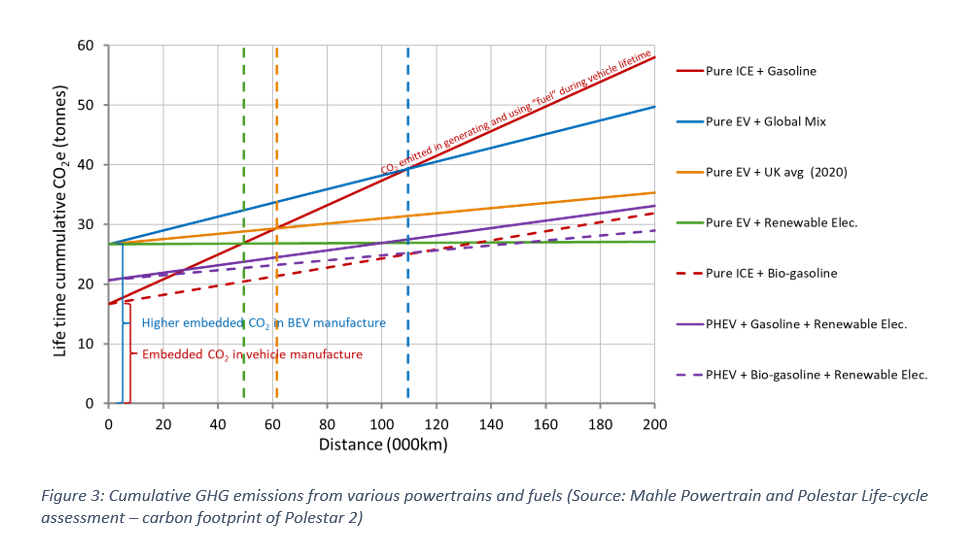
Final Thoughts
Firstly and as the figures above show, there is no doubt that the overall life-cycle emissions for a pure BEV will be less than a conventional, ICE-only vehicle running on fossil fuel (assuming the battery pack does not need replacing in the vehicle lifetime) and it is absolutely correct that we should be following this policy.
However, as we have shown, focusing only on tailpipe emissions will appear to reduce CO2 emissions at a local or national level, but risks missing key CO2 emissions from elsewhere. Global warming potential depends not only on the amount of CO2 emitted but also how long it is in the atmosphere and so we should recognise that the manufacture of the battery packs for EVs effectively accelerates CO2 emissions in the short-to-medium term.
Secondly, plug-in hybridisation and the use of sustainable fuels are equally valid means of reducing CO2 emissions and the latter is the only method we have at our disposal to reduce those emissions from the vehicles currently on the road. As a result, this technology should be incentivised at least as much as electrification.
EVERYTHING IS POSSIBLE. AND IT STARTS WITH A CONVERSATION.
GET IN TOUCH